Conference report: ASME 2014 ICE Fall Technical Conference
30 October 2014
The ASME Internal Combustion Engine Division 2014 Fall Technical Conference was held on October 19-22, 2014 in Columbus, Indiana, USA and was hosted by Cummins. The two-day technical program included more than 100 technical papers. The conference was attended by about 260 delegates.
Please log in to view the full version of this article (subscription required).
Keynote Lecture. The conference opened with a keynote presentation by John Wall, Cummins Chief Technical Officer on the evolution of emission control for heavy-duty diesel engines from the 1990s. Prior to this period, there were a variety of engine platforms available for heavy-duty engines including: four-strokes, two-strokes, naturally aspirated, turbocharged, two valve/cylinder, and four valve/cylinder. In the 1990s when stricter emission limits came into place, there was shift to a common set of technologies from all manufacturers of heavy-duty engines including: 4-stroke, 4 valves/cylinder, turbocharged, aftercooled and electronic fuel injection. This approach of using a common platform, with a few exceptions, continued into 2004 with the introduction of EGR engines, 2007 with the introduction of DPFs and 2010 with the introduction of urea SCR systems.
One notable exception to the common platform approach from Cummins was the Dodge Ram pick-up that used a NOx adsorber to meet the 2010 heavy-duty NOx limit starting in 2008. While NOx adsorbers were touted as a potential solution for heavy-duty engines when the 2007/2010 limits were promulgated, they were never able to provide sufficient performance at high exhaust temperature to be a serious contender to meet the 0.2 g/bhp-hr FTP NOx limit for heavy-duty engine certifications. However, by making use of an alternative certification option, Cummins was able to use the light-duty chassis certification procedure with its relatively low exhaust temperatures ideally suited for a NOx adsorber to fully comply with the 2010 heavy-duty legal requirements and generate significant NOx credits.
As the focus for heavy-duty engines shifts toward lowering CO2 emissions and fuel consumption, heavy-duty engines will continue to evolve. Friction reductions and advanced combustion technologies are two important areas specific to the engine that will see considerable focus. Other technology areas include low carbon fuels, better integrating engine and transmission to enable downspeeding and aftertreatment systems that work better at low exhaust temperatures.
Waste heat recovery (WHR) will be an important technology that could increase engine brake thermal efficiency (BTE) by 4-5%. Cummins focus for WHR is on line-haul truck applications that run at relatively constant speed. Hybrid drivetrain technologies would be better suited than WHR for applications that operate over more transient cycles. Typically, the closed loop WHR systems have an efficiency of about 20%. Cummins currently has 3 trucks equipped with WHR in operation that have accumulated a combined total of over 80,000 miles. One of these is the Peterbilt/Cummins SuperTruck that was on display during the conference.
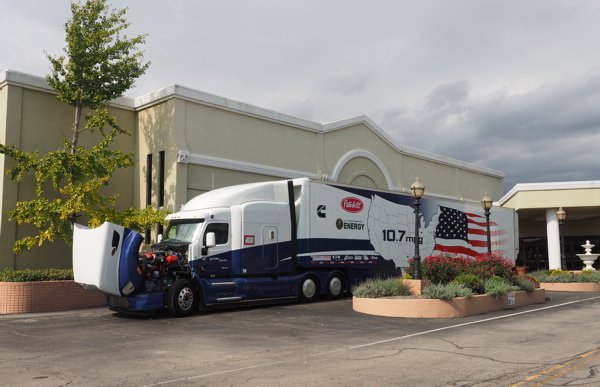
The Cummins WHR system uses an axial flow WHR turbine and a low global warming potential (GWP) organic refrigerant. A WHR system boiler replaces the EGR cooler on the engine. The turbine output shaft is connected via a speed reducing transmission to the engine’s front drive belt. As reported earlier this year at the DOE Merit Review, the WHR is able supply almost 15 hp. VanDyne SuperTurbo, Inc. has been a participant in the Peterbilt/Cummins SuperTruck program since the beginning and most likely provides the high speed transmission for the WHR system.
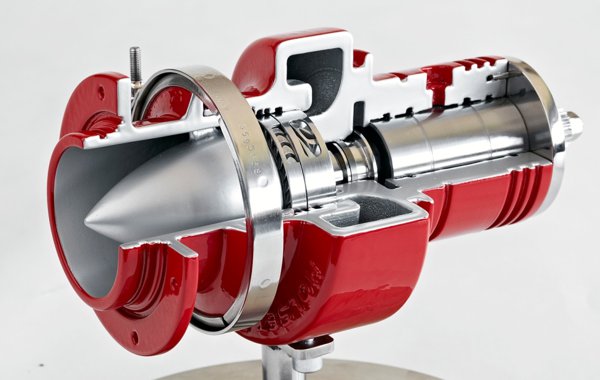
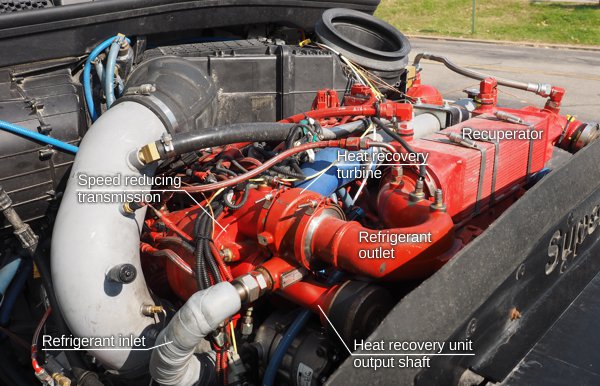
Waste heat recovery is an important tool that has allowed Cummins to meet some important SuperTruck goals. They have already met the goal of demonstrating a 50% BTE engine (they achieved 51% BTE) at typical highway cruise conditions for a line haul truck and demonstrated significant improvements in freight efficiency. They have achieved 10.7 MPG from a loaded class 8 tractor/trailer. The break-down of where the freight-efficiency improvements come from is as follows: 41% engine, 44% vehicle aerodynamics and 15% from the driveline and tires.
Natural Gas & Alternative Fuel Engines. With natural gas prices in North America predicted to remain significantly below oil prices, natural gas—CNG and LNG—continues to be a potentially attractive fuel for a wide range of engine applications.
Southwest Research Institute (SwRI) reported on an experimental study of a dual fuel engine that explored the limits of natural gas substitution [ICEF2014-5476]. A Navistar MaxxForce 13 diesel engine was used, with natural gas fumigated into the intake air. The engine was operated at a lean and an enriched equivalence ratio and tested over the ESC cycle. Gas substitution ratios from 10 to 95% were investigated. Increasing substitution rates were found to cause slower flame propagation, require advanced timing and lead to lower peak cylinder pressures. Emissions of methane/GHG increased with natural gas, especially at lower engine loads, while NOx emissions were reduced at elevated gas substitution rates. The study also found that the factory fuel injectors were difficult to use at the narrow injection pulse widths that were needed at high gas substitution rates. Injectors optimized for pilot service would be necessary for higher NG substitutions.
Natural gas engines can only produce GHG emission benefits if there are no leaks of methane—a gas with a high GWP. A number of prior studies found that if methane leaks approach 5% of the total gas volume, natural gas provides no GHG emission benefit compared to petroleum fuels. It is still very uncertain what the overall methane leaks during gas production, distribution and use are. An interesting study by West Virginia University quantified methane emissions from the engine units in five gas compressor facilities [ICEF2014-5626]. Methane leaks (defined as an unintended malfunction) and losses (defined as a design feature) were measured on-site from six types of engines, including two- and four-stroke engines as well as gas turbines. Methane emissions from particular engines, relative to the fuel consumption of the engine, ranged from 0.5% (Clark TLA-6) to 7.6% (CAT 3512). Overall, for the five sites tested, the engine and compressor units yielded a combined methane leak and loss rate of 71.1 kg/hr. The highest source of CH4 emissions was engine exhaust, contributing 61% of the total emissions. Other sources included compressor packing loss and wet seals in turbines (25%), engine leaks (7%) and crankcase leaks (7%).
Locomotives. With US Tier 4 locomotive emission requirements going into effect from January 2015, several talks discussed the development of Tier 4 locomotive engines, including papers by GE and Cummins (Caterpillar’s EMD announced earlier it will not offer Tier 4 engines in 2015). Cummins presented two papers on their new, QSK95 high speed locomotive engine platform: one dealing with the combustion system development [ICEF2014-5517] and another about the impact of air humidity on NOx emissions, urea (DEF) consumption and the sizing of the urea tank [ICEF2014-5526]. The development of the QSK95 engine was supported with combustion analysis using STAR-CD and GTPower software to optimize various design parameters, such as swirl, bowl shape and nozzle configuration. The design was validated using a single cylinder test engine. The emission approach chosen by Cummins involves in-cylinder PM emission control (e.g., full load smoke was reduced by 50% through a fuel injection strategy) and SCR aftertreatment for NOx control. The SCR system, utilizing a vanadia SCR catalyst and a feed-forward control based on engine-out NOx, provides over 90% NOx reduction. To aid future customers in specifying the size of urea tank for their applications, Cummins conducted an experimental study on the impact of air humidity and temperature on NOx emissions and urea consumption. The impacts were found to be significant—moving the locomotive from operation at “standard humidity” of 10.71 g/kg to operation with dry air (0 g/kg) would require increasing the volume of urea tank by more than 30%. The QSK95 engine is likely to be first launched in a passenger locomotive application.
Tier 4 locomotives from GE—a major supplier of freight locomotives—will meet the emission requirements without exhaust gas aftertreatment. The GE emission and engine efficiency strategies are believed to include EGR and Miller valve timing. GE presented a paper evaluating four alternative EGR strategies for Tier 4 locomotive engines [ICEF2014-5552]. The EGR methods (all of which were high pressure loop EGR) included: (1) backpressure, such as exhaust gas throttling, (2) donor cylinders, where two cylinders on the12 cylinder engine are dedicated EGR suppliers, (3) EGR pump, and (4) turbocompounding. Backpressure was the most costly method in terms of efficiency, with a fuel consumption penalty of 3-4%. Turbocompounding, on the other hand, provided about 2% fuel consumption benefit, but was deemed complex and expensive. While GE has not revealed their final choice of technology, it appears to be either donor cylinders or the EGR pump.
Hybrid Buses. Several papers by Cummins on hybrid urban bus programs illustrated the different approaches that must be used for these vehicles in different parts of the world and the difficulty of having a single global hybrid bus platform. In the UK, incentives through the Bus Service Operator Grants (BSOG) require that buses be certified by the Low Carbon Emissions Board (LCEB) to qualify for a subsidy. One requirement for certification is that the bus provide 30% lower CO2 emissions relative to a fleet of Euro III buses operating over a drive cycle based on a particular London bus route. To meet this objective, Cummins chose a series hybrid architecture with energy management being a combination of charge-depleting and load-following strategies depending on engine operating conditions. The selection of engine operating points suitable to meet the CO2 reduction requirements while maintaining maintain Euro VI emission limits in charge-depleting mode leads to a more restrictive operating envelope than would be the case for Euro V and earlier hybrid buses. In addition to keeping BSFC low and maintaining low steady-state NOx, the additional considerations for Euro VI included maintaining passive DPF regeneration, maintaining aftertreatment system temperature, meeting NVH requirements and OBD requirements. These considerations lead to the selection of an engine operating envelope below about 40% load and relatively far from the minimum BSFC point of the engine selected for this application. For load following operation, additional considerations for engine operating points included drivability and smooth delivery of power. An important measure to keep fuel consumption low during periods when air-conditioning is required was to use an electrically driven compressor to avoid the need to continuously run the engine. Hybrid operation also requires some additional considerations for the engine due to start/stop effects: the fuel system seals needed to be more robust to prevent fuel leaks due to more frequent pressure spikes, poor lubrication of crankshaft bearings due to oil pressure delay was dealt with by using bearings with a polymer overlay that could cope up with friction during the time it takes for oil to reach the bearings, an idle latch time was implemented to avoid overheating turbocharger bearings due to hot shut-downs, harmful engine stops were avoided by preventing an engine stop if the coolant temperature is too high.
Technical Tours. On the third day of the conference, participants could attend tours of the Cummins Technical Center, Cummins Fuel System Plant, Cummins Mid-range Engine Plant and the Seymour Engine Plant. The Cummins Technical Center is the worldwide technical headquarters for Cummins. Cummins Fuel System Plant manufacturers high pressure common rail fuel system components and injectors. Cummins Mid-range Engine Plant manufacturers the 6.7 L engine. The Seymour Engine Plant manufactures high horsepower engines including the QSK19, QST30 (in a joint venture with Komatsu) and the V903 military engine. Expansion of the Seymour Engine Plant to manufacture the new QSK95 engine is currently underway.
Conference website: www.asmeconferences.org/ICEF2014