US DOE Merit Review 2020—aftertreatment technologies
11 August 2020
The US Department of Energy (DOE) Vehicle Technologies Office (VTO) 2020 Annual Merit Review (AMR) included a number of presentations reflecting trends in future aftertreatment technologies. A principal goal of future low temperature aftertreatment technologies is to achieve greater than 90% conversion of criteria pollutants (NOx, CO, HCs) at 150°C for the full useful life of the vehicle. Additionally, these technologies must optimize or replace precious metal usage to control cost and minimize the production of N2O and CH4 to avoid potential negative adjustments to fuel economy ratings [4846][4847].
Please log in to view the full version of this article (subscription required).
Selective Catalytic Reduction (SCR)
Incorporating an SCR catalyst onto a DPF is a significant challenge for heavy-duty applications because of several challenges including the potential loss of passive soot oxidation and the resulting implications for fuel consumption. One approach to address this challenge being pursued by PNNL and PACCAR is the application of a selective catalytic oxidation (SCO) phase to the SCRF that will promote NO oxidation to NO2 while minimizing the oxidation of NH3 [4833]. The SCO phase selected is 10% Mn/ZrO2 applied to a Cu/SSZ-13 SCR catalyst and has been demonstrated to favorably shift the NO2/NOx balance with no additional high temperature NH3 oxidation. Additionally, low temperature SCR activity improved and N2O emissions were not adversely affected. For passive soot oxidation, the SCO shifts the pressure drop profile to temperatures about 30°C lower while shifting the CO2/CO balance of the effluent towards CO2. Sulfur durability and desulfation requirements are still to be determined.
With proposed increases in the full useful life requirements by CARB and EPA for on-road heavy-duty diesel engines, there is a pressing need to not only better understand catalyst aging but to develop more durable catalysts. To this end, Oak Ridge, Cummins, Johnson Matthey are applying transient response Cu redox half cycle analysis [4834] to better understand the field aging process of Cu-SCR (Cu-SSZ-13) catalysts and its impacts on low-temperature performance [4835]. This analysis technique measures the shape of the conversion inflection immediately after the SCR catalyst is “turned on” to determine the catalyst’s kinetic parameters. Early results show that field aging is not replicated by hydrothermal aging (HTA) in the lab—field aging results in Cu-site loss that does not appear in HTA aging. Temperature-programmed desorption (TPD) experiments showed that desorption of ammonia from a HTA sample decreases at high temperature and increases at low temperature relative to a degreened catalyst sample. For the field aged sample, high temperature ammonia desorption decreased but the low temperature desorption increase was much lower. Along the same lines, PNNL is analyzing hydrothermally aged Cu/SSZ-13 SCR catalysts using Electron Paramagnetic Resonance (EPR) and has observed the formation of more stable and less active Cu(II)-NH3 intermediates with HTA that reduce SCR activities [4836].
Methane Catalysts
Work at PNNL, in conjunction with Oak Ridge, GM and Johnson Matthey, is apply atom trapping [4839] to create thermally durable Pd single atom catalysts with reduced PGM usage that will improve the low temperature performance of methane oxidation catalysts [4840]. The goal is to achieve >95% methane conversion at <350°C without detrimental effects from sulfur and water. So far the work has confirmed that PdO clusters (<2 nm) and Pd nanoparticles (>5 nm) are 2-4 orders of magnitude more active than atomically dispersed ionic Pd2+ for methane conversion at 450°C. However, they exhibit similar or lower activity on SSZ-13 than on alumina, suggesting no apparent advantages if PdO clusters and Pd nanoparticles are used with zeolite supports. Further investigation showed that PdO clusters deactivate due to coverage by Al2O3 formed via H2O induced hydrolysis of Al3+. Supports with low Al content showed much lower deactivation. While 3% Pd + 0.5% Rh on a CeO2 support shows 90% conversion at about 350°C, further work is required to reduce water inhibition at low temperatures, improve catalyst cyclic stability, thrift the precious metal usage and mitigate catalyst deactivation by sulfur.
For stoichiometric natural gas engines, work at the University of Houston (supported by CDTi Advanced Materials, Inc., University of Virginia and Oak Ridge) suggests that spinel group minerals in conjunction with PGM loadings of 30 g/ft3 could provide significant improvements in methane conversion over TWCs [4841][4842]. Using a formulation containing 30 g/ft3 PGM/100 g/L spinel (Mn0.5Fe2.5O4), a significant reduction in light-off temperature was achieved compared to a 30 g/ft3 PGM catalyst with no spinel, Figure 1. In catalysts with spinel, the lower light-off temperature is only apparent if the air fuel ratio (AFR) is modulated. While modulation also improves the conversion over the 30/0 catalyst, the spinel still provides about a 50°C further reduction. Testing with SO2 in the feed gas suggests that spinel mitigates the detrimental effect of sulfur poisoning at selected AFR modulation frequencies. Spinel addition is claimed to provide a more favorable partially oxidized PGM at an AFR closer to stoichiometric that results in higher methane conversion and lower selectivity to NH3 production.
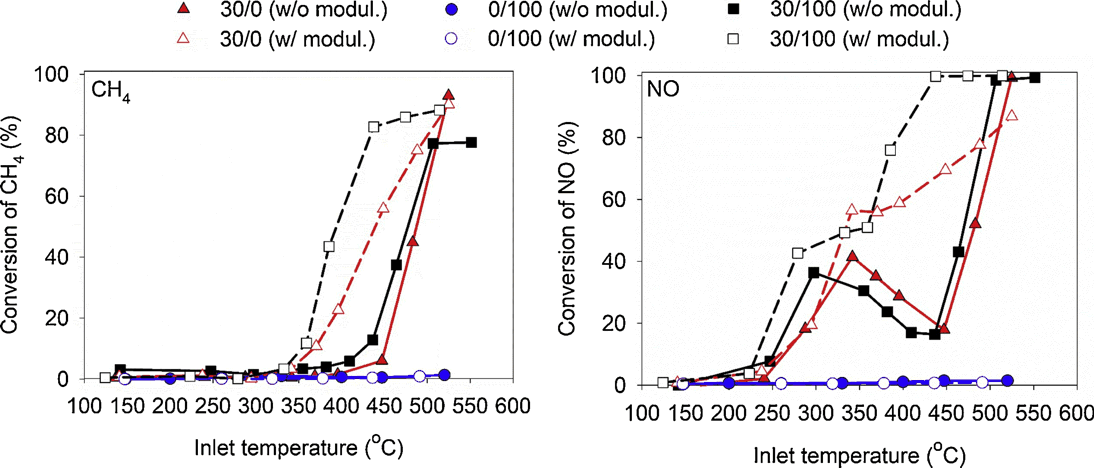
Other Topics
Other aftertreatment developments include a passive SCR system being developed by Oak Ridge, GM and Umicore [4843]. An aftertreatment system for an engine designed to operate in lean burn mode under most drive cycle conditions and stoichiometric operation elsewhere is shown in Figure 2. A Pd-only TWC would be used to generate ammonia via rich pulses while a second TWC having a NOx storage function would store NOx during lean operation. Engine tests demonstrate that NOx+NMHC below Tier 3 bin 30 levels are possible but CO emissions and NH3 utilization remain as challenges. Secondary air injection and/or a clean-up catalyst may be required. Aging reduces the NH3 storage capacity of the SCR catalyst and increases NOx emissions – especially under stoichiometric and rich conditions.
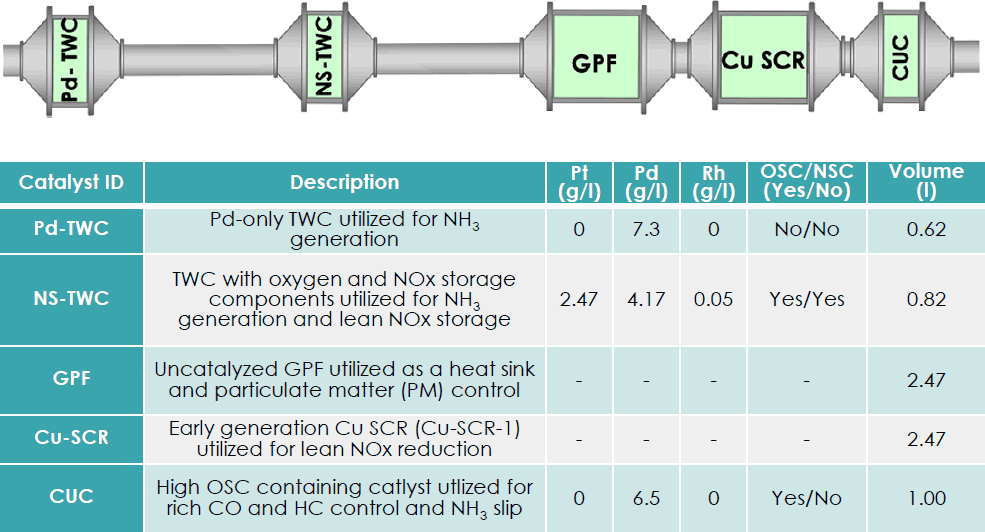
Another program at the University of Houston with cooperation from the University of Virginia, Oak Ridge, Johnson Matthey, SwRI and FCA is designing a combined hydrocarbon/NOx trap for lean burn engines such as diesels that also incorporates a low temperature oxidation catalyst [4844]. Pd based zeolite (Pd/H-SSZ-13, Pd/H-ZSM-5, Pd/H-BEA, Pd+Fe/ZSM-5 and Pd+Cu/SSZ-13) formulations are being examined for the PNA. For the hydrocarbon trap, H-BEA zeolite would trap diesel-like hydrocarbon at lower temperature while for lighter hydrocarbons, Ag+ exchanged H-ZSM-5 shows enhanced uptake. A Pt/Al2O3 component would catalyze hydrocarbons upon release at higher temperature. Two new oxidation catalysts for low temperature oxidation are being developed: a Cu, Ce, Co mixed-metal oxide + Pt/Al2O3 and a PdCu alloy/SiO2 + Pt/Al2O3.
A program at the University of Kentucky with Ford, Oak Ridge, Purdue, UC Berkeley and BASF is developing a passive HC/NOx trap for low temperature gasoline applications [4845]. Work has focused on studying Pd/CHA and Pd/BEA for NOx storage. Pd/CHA was determined to be more resilient to reduction than Pd/BEA. On-going work is exploring how the Al distribution in CHA impacts NOx storage capacity and catalyst durability—a number of CHA samples with different amounts of paired Al sites have been prepared.