Conference report: ASME ICE 2018 Fall Technical Conference
29 November 2018
The ASME Internal Combustion Engine 2018 Fall Technical Conference (ICEF 2018) was held on November 4-7, 2018 in San Diego, California. The conference was attended by about 260 delegates and hosted by Achates Power—a developer of two-stroke, opposed piston engines. The event included a two-day technical program with 110 technical papers, as well as keynote talks, and student presentations. After the Conference, the participants could take part in a technical tour of the Achates Power facilities in San Diego.
With much talk recently of banning internal combustion engines, many are left wondering if the ICE is a dying technology. However, as David Johnson, President and CEO of Achates Power pointed out, even if ICEs disappear from the light-duty market by 2050, at least 3 billion more ICEs will be needed. This leaves plenty of opportunity to develop them further. One particularly challenging application is light-duty pickup trucks where significant improvements in fuel economy to meet fuel economy targets in 2020-2025 (neglecting for the moment the potential for any rollbacks) could be very challenging and costly.
Achates power thinks their opposed piston engine may provide a cost effective solution that could provide an unadjusted CAFE fuel economy of 37 mpg with gasoline (gasoline compression ignition - GCI) and 42 mpg with diesel on a Ford F-150 platform; a 29% improvement over the 2.7L Ecoboost currently available for this vehicle. For the EPA fuel economy label, the estimated values are 25/32 mpg city/highway for GCI and 28/37 for diesel.
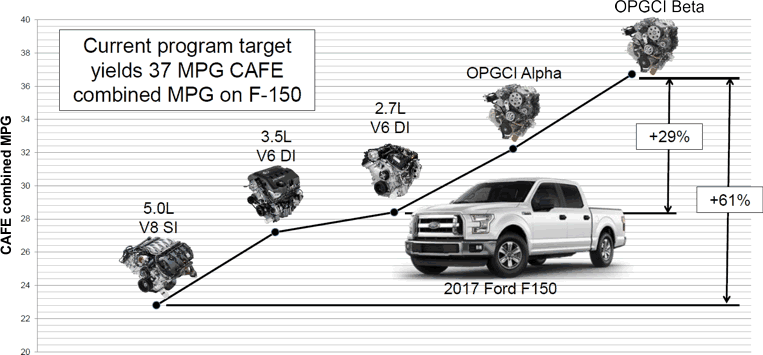
Some key features of the opposed piston engine that allow it to achieve high efficiency include: reduced heat transfer, earlier and faster heat release and reduced pumping work. The ability to vary the quantity of trapped residual gas also allows stable gasoline compression ignition and higher exhaust temperatures for rapid catalyst light-off. The cost effectiveness arises from a reduced part count, smaller displacement (it’s a 2-stroke) and smaller, less expensive aftertreatment and it can be manufactured using conventional processes and materials. It also fits existing vehicle platforms, has competitive NVH characteristics and is compatible with hybrid drivetrains. A number of challenges have been addressed including oil consumption which currently averages about 0.05% of fuel consumption; similar to modern 4-stroke engines. Further details on the Achates engine program for light-truck applications is provided below.
Achates Power has a number of engine programs with different manufacturers including a 10 L/450 hp program with Peterbilt for heavy-duty trucks, a 14 L/1000 hp program with Cummins for military combat vehicles and a 203 L/5000 hp program with Fairbanks Morse for industrial applications. The later was announced in 2013 as a joint development and licensing agreement to reduce emissions and fuel consumption of Fairbanks Morse diesel and dual-fuel opposed-piston engines. A new Tier 4 final engine from Fairbanks Morse, announced late last year, is a result of this cooperation.
Natural Gas/Dual Fuel Engines
This years conference featured numerous papers relevant to natural gas engines; both dual fuel and spark ignited.
In follow-up work to that presented last year, Technical University of Munich researchers examined the processes prior to ignition relevant to dual-fuel natural gas engines using Miller intake valve timing [ICEF2018-9671]. Miller valve timing leads to low TDC temperatures, longer ignition delay, deeper penetration and higher dilution of the diesel pilot spray. The results showed that the autoignition of the pilot fuel strongly depends on the fuel concentration within the spray. High-speed shadowgraph images revealed that in the case of very low fuel concentration within the pilot spray only the first-stage of the two-stage ignition occurs, leading to large cycle-to-cycle variations and misfiring. However, reducing the number of injection holes counteracts this effect. Compared to a diesel injector with 10-holes, a 5-hole injector showed shorter ignition delays, more stable ignition and a higher proportion of ignited sprays.
The Austrian Large Engines Competence Center (LEC GmbH) evaluated a number of strategies to improve the transient performance of dual fuel engines in genset applications [ICEF2018-9710]. Rapid load acceptance in gas fueled engines can be challenging because of knock considerations, making it difficult to meet some of the performance requirements of ISO 8528-5. Dual fuel engines that have the potential to operate in full diesel mode during load acceptance can significantly improve the load acceptance rate. The limiting factor of load acceptance in the engine studied was the need to avoid knock while gas was purged from the intake system during the transition to 100% diesel operation; gas was supplied from a single mixer at the turbocharger compressor inlet. Further investigation with port injection of natural gas and variable valve timing are planned to further improve load acceptance.
In another project, LEC GmbH along with ABB Turbo System Ltd. and GE Jenbacher GmbH examined the potential of variable intake valve actuation to improve engine efficiency and performance at off-design conditions [ICEF2018-9523]. Compared to engines with fixed valve timing and lift, variable valve train offers the potential to accommodate changes in ambient temperature and pressure as well as changes in the methane number (MN) of natural gas. By using the variable valve train rather than a compressor bypass to control power, variable valve actuation results in a more favorable pressure drop (Pexhaust-Pintake) across the engine and can also allow the use of the Miller effect to manage in-cylinder temperature. A 0.6% increase in BTE was estimated at design conditions using the variable valve train with the benefit increasing as ambient temperature decreased. The BTE of the variable valve train engine remained higher as ambient pressure decreased (altitude increased) and fuel MN decreased.
GE Global Research Center examined natural gas substitution rates of 0, 60 and 93% (by energy) in a single cylinder engine with GE Evolution locomotive power assembly [ICEF2018-9765]. The diesel injector was sized to allow 100% diesel operation. For the 60% substitution, injection timing was matched to diesel and NOx reductions but little efficiency benefit was noted. For 93% substitution, diesel injection occurred early (40°BTDC and earlier) and tested values spanned the range between misfire and knock. Combustion with 93% natural gas was “RCCI-like” and offered significant NOx reductions (~85%) while increasing relative efficiency up to 9%. However, combustion variability was very high due to variations in fuel injection quantity; the injector operated entirely in the ballistic region at these low fuel injection quantities.
Canada’s National Research Council (NRC Canada) examined the effect of natural gas fractions from 0 to 90% on the diesel injector tip temperatures in their single-cylinder research engine based on a Caterpillar 3401 [ICEF2018-9664]. The work was driven by concerns that when replacing diesel with natural gas in dual fuel engines, the diesel injector tip may overheat and lead to nozzle coking. While increases in injector tip temperature up to about 25°C were noted with increasing natural gas fraction at a given diesel injection timing and with advancing the diesel injection timing at a given natural gas fraction, the injector tip temperature never exceeded 250°C.
The University of Pisa carried out a numerical investigation on the effect of port and direct injection of CNG and LNG on volumetric efficiency in SI engines [ICEF2018-9532]. Direct injection of CNG before the inlet valves close gave the lowest volumetric efficiency because it hindered the entry of air. Injection of LNG into the intake manifold before the intake valves closed maximized the trapped volumetric efficiency and therefore the engine power.
Retrofitting older 2-valve stationary natural gas engines with a 4-valve head design can improve electrical efficiency up to 2.5% as demonstrated by PGES GmbH [ICEF2018-9646]. This can raise the electrical efficiency to 42.9% primarily through lower gas exchange work. By comparison, modern state-of-the-art 4-valve engines achieve electrical efficiencies of about 45%.
Generac discussed the development of a new, 4.5 L spark-ignited engine for power generation [ICEF2018-9616]. Generac designed a 9 L V8 engine for generators of 80-100 kW (naturally aspirated) and 130-150 kW (turbocharged). The engine was launched in 2012, and then redesigned in 2017. Based on the cylinder head and power cylinder of the V8 unit, a 4.5 L inline four cylinder engine has been designed. The engine can support generators of 42-50 kW (naturally aspirated) and 60-80 kW (turbocharged), and can be operated on natural gas and LPG.
Engine Efficiency
Professor Chris Edwards from Stanford provided his thoughts on high efficiency engines during a lunchtime talk on the second day. One of the paths being pursued to high efficiency is through combined cycles such as coupling the internal combustion engine to a Rankine bottoming cycle and perhaps a solid-oxide fuel cell. If this combined cycle approach is combined with a low heat rejection “diesel” engine fueled with methanol and operating rich (around ɸ=2), about 70% exergetic efficiency is possible, figure below. The rich equivalence ratio reduces combustion irreversibility in the engine and using methanol even at these rich conditions yields very little soot. However, the level of complexity is significant and even higher efficiencies would be impractical with this approach; there are too many small losses that would each have to be reduced a small amount to achieve further significant gains. An efficiency of 80% is thought to be possible but would require a significant deviation from the linear piston/crank mechanism to achieve the extreme compression/expansion ratios required for a simpler approach. Spherical expansion was mentioned as one possible approach; volume changes would be proportional to changes in length cubed (dV ~ dL3) and would help keep the system compact. How this could be achieved was not clear.
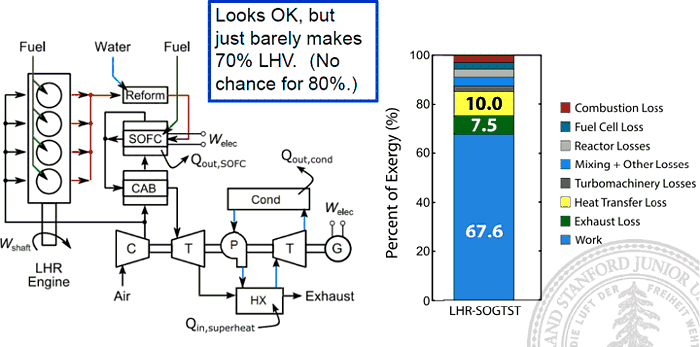
UC Berkeley is proposing a closed loop internal combustion engine cycle working with a monatomic gas (argon in this case) and using a membrane gas separation unit [ICEF2018-9670]. A synthetic mixture of monatomic gases and oxygen yields a theoretical thermal efficiency of up to 80%, more than 20% higher than air cycles. With a hydrocarbon fuel, a gas membrane would also be required to remove CO2 from the combustion products; facilitating its sequestration. Experiments were carried out with a CFR engine to verify the efficiency gains with Argon as a working fluid - a maximum indicated thermal efficiency of about 43% was achieved with Argon/O2 mixtures compared to 36% with air using methane as the fuel. While the experiments did not test gas separation membranes, numerical simulation suggested an energy penalty of 2% to capture all of the CO2 emissions.
FEV developed a vehicle-level model to evaluate the functionality of their integrated turbocompounding, electrification and supercharging (ITES) approach [ICEF2018-9703]. The system manages the power flow between the turbocompound turbine, secondary compressor, 48V electric motor/generator, and the engine by employing a planetary gear set. The vehicle integration model was based on a Class 6-7 vocational truck, where the basic 7.7 L, 6 cylinder engine was downsized to 5.1 L, 4 cylinder engine. The system also included a 15 kW motor/generator, a 20 kW turbocompound turbine, and a 5 kW secondary compressor. The vehicle showed a 11.1% increase in fuel economy (CARB GHG Phase 2 cycle). The primary contribution to this increase came from engine downsizing (6.7%), followed by start-stop (2.4%), turbocompounding (0.9%), regenerative braking (0.7%), and electric water pump (0.5%).
Advanced Combustion
A number of advanced combustion papers focused on gasoline compression ignition (GCI). Several papers were given on 2-stroke opposed piston (OP) engines, fueled by both diesel and gasoline—in the latter case, primarily as GCI (OPGCI). The OP engine architecture eliminates the cylinder head of a conventional engine, thus reducing the surface area-to-volume ratio, reducing heat transfer losses and increasing thermal efficiency.
Initial results from steady-state testing on a new 2.7 L OPGCI multi-cylinder engine for a pick-up truck application were presented by Achates Power [ICEF2018-9610]. The engine has 3 cylinders, a compression ratio of 18.5:1, a power output of 200 kW, and 650 Nm of torque. The intake charge system includes a variable geometry turbocharger, a supercharger, and a high pressure EGR. The fuel injection system utilizes Delphi Technologies injectors, 2 per cylinder, and two common rails, where each rail can be run at a different pressure. The engine has been designed to fit into a F150 light-duty truck. The initial test results showed a 31.7% BTE (cycle average over LA4 cycle), high combustion efficiency, similar to diesel, even at low load, and a 365°C exhaust gas temperature while producing only 1 g/kWh NOx. The final target is 36.5% BTE, a 30% improvement over conventional 4-stroke engines. A simulation using an off-the-shelf diesel aftertreatment showed Tier 3 Bin 160 emissions. Tier 3 Bin 30 emissions are expected with gasoline fuel and aftertreatment. An additional pre-turbo catalyst may be needed to reduce CO/HC emissions. A computational fluid dynamics (CFD) simulation to develop the OPGCI engine was conducted in cooperation with Argonne [ICEF2018-9713].
Another study by Achates Power compared diesel and GCI combustion and emission performance in a single cylinder 2-stroke OP engine [ICEF2018-9701]. It was found that GCI could be operated over the same load range as diesel, and offered a similar thermal efficiency. GCI achieved over 98% combustion efficiency—higher than 4-stroke engines and almost as good as diesel. GCI was able to use lower EGR rates to reach the same NOx emissions as diesel, and had significantly lower soot emissions. However, GCI had increased HC and CO emissions from the larger and earlier pilot injection compared to diesel, and had a higher combustion noise.
University of Bath and Saudi Aramco examined three different uniflow scavenging options for a medium-duty 2-stroke engine for a US light-truck application [ICEF2018-9766]. Engine designs using opposed-pistons, a poppet valve with piston-controlled intake ports and a sleeve valve were considered. The opposed-piston configuration provided maximum expansion and minimum heat transfer leading to a net specific fuel consumption (NSFC; ISFC net of supercharger power) benefit of 8.3%. The opposed piston design also required minimum air supply system work, included in this NSFC value. The poppet-valve uniflow approach was limited by the kinematics of the valve train system. The sleeve-valve design had the best potential for breathing at higher engine speeds due to the absence of kinematic limitations but its limiting factor was found to be the interaction between piston and sleeve motion near BDC.
Michigan Technological University and Hyundai conducted a numerical investigation of a 6-stroke GCI engine with continuously variable valve duration (CVVD) control [ICEF2018-9590]. The 6-stroke cycle included another compression stroke (exhaust valve closed), a second injection and a second power stroke. The concept allows recovery of some of the heat wasted in the 4-stroke cycle. In the 6-stroke cycle, fuel was divided evenly between the 1st and 2nd power stroke. Both soot and NOx emissions were found to increase in the 6-stroke cycle. Valve timing strategies were investigated to control the process.
Partially premixed combustion (PPC) in a multi-cylinder engine with the Volvo Wave piston was studied by Lund University [ICEF2018-9568]. A Volvo D13 heavy-duty, single-stage VGT engine with the Wave piston was run at medium and high engine load using PRF70 fuel (70% iso-octane and 30% n-heptane) and Swedish MK1 diesel. Engine performance and efficiency were similar with both fuels. Peak gross indicated efficiencies were around 49% at 1000 Nm and slightly above 50% at 2000 Nm—lower than the 55% efficiency found earlier in a single cylinder engine. NOx emissions of 1 g/kWh were obtained, while complying with Euro VI PM limits. However, once the engine-out NOx was lowered closer to the Euro VI NOx limit, the low soot emission performance could not be sustained and PM quickly increased above acceptable levels.
Spark-Ignited Engines
Wayne State studied different approaches to detect misfiring, knock and sporadic pre-ignition in production direct injection gasoline engines [ICEF2018-9589]. They examined a multi sensing fuel injector (MSFI) that senses ion current, a standalone ion current probe, and a spark plug applied to act as an ion current sensor. These sensors can give more information about combustion than a knock sensor. The MSFI and spark plug sensors would be most feasible since they wouldn’t require an additional opening in the cylinder head. Start of combustion can be detected only by the spark plug/ion sensor if the noise in the signal produced from the ignition system is eliminated. The signals from the standalone sensor and MSFI occur later than the signal produced by the spark plug ion sensor.
Mahle discussed the development of a test procedure for evaluating Low Speed Pre-Ignition (LSPI) in SI engines [ICEF2018-9760]. The test is based on a 270 kW/550 Nm V6 GDI engine, is about 3.5 h long and consists of 6 repetitions of 25 minutes of full load at 1700 rpm/5 minutes idling. Oil aging increased LSPI propensity; possibly due to wear metals or changes in oil properties such as TBN or due to oxidation. Ethanol blended fuel reduced the probability of observing LSPI events. Several issues were observed that are not fully understood including: an decrease in LPSI propensity with engine aging and poor engine-to-engine repeatability.
The University of Michigan has been studying ways to control SI engines at EGR rates near the limits of combustion stability [ICEF2018-9540]. At these conditions, minimizing the occurrence of misfires and partial burns is critical to maintain combustion stability. However, based on their analysis, it may not be necessary to observe both; the observation of only partial burns may be sufficient to control the upper bound on the probability of misfire events. The observation of partial burns has an advantage in that it requires a smaller estimation window.
Diesel Engines
Universitat Politècnica de València and HORIBA tested a diesel passenger car at altitude and with HORIBA’s altitude simulator [ICEF2018-9549]. They found that the turbocharged diesel engine performance is limited at increased altitude primarily to protect the turbocharger compressor; it approaches temperature, surge and over speed limits with increasing altitude. Some strategies to protect the turbocharger compressor include reducing full load EGR with altitude and eventually shut-off EGR completely above 1300 m. However, this increases the stress on the turbine whose inlet temperature limit is then more easily reached at high altitude. Reducing the full load torque values especially at high engine speeds can limit turbine inlet temperature.
The University of Michigan and Ford discussed a control approach that could potentially maximize the capability of using retarded fuel injection timing for exhaust ATS thermal management [ICEF2018-9638]. Over-retarding injection timing can lead to vibration and harshness resulting from increased combustion variability. They propose a closed loop controller for aggressive injection timing retard to maximize ATS heating using and in-cylinder pressure sensor. Windowed statistical feedback is used to maintained IMEP variation at an acceptable limit.
In another project, the University of Michigan and Ford found that the flow field of LP-EGR and air mixing upstream of compressor inlet can affect compressor performance through introduction of prewhirl or the perturbation of the velocity triangle at the turbocharger compressor impeller inlet [ICEF2018-9686]. Manipulation of this flow field introduces another pathway for improving compressor performance in diesel engines.
Wayne State modeled diesel fuel injector nozzle erosion due to cavitation [ICEF2018-9553]. Using a cylindrical nozzle hole, higher pressure drops decrease surface erosion from cavitation since the location of bubble collapse is shifted toward the nozzle center and exit.
FEV has carried out a determination of the minimum cylinder displacement for a 4-stroke diesel engine, given the limitations posed by state-of-the-art technology and while maximizing power density [ICEF2018-9522]. Current diesel engines are have cylinder displacements of 200 cm3/cylinder and higher while gasoline engines can be as small as 25 cm3/cylinder. A preliminary layout of 95 cm3/cylinder, 2-valve design was identified. The bore is limited to 55 mm to maintain an acceptable intake valve flow coefficient. Restricting the bore-to-stroke ratio to 0.7-0.8 to allow high speed operation gives a stroke about 40 mm. To achieve a power density of at least 50 kW/L, boosting is required. The smallest turbocharger available requires four cylinders at 95 cm3/cylinder.
Alternative-Fueled Engines
A project funded by the Netherlands Organisation for Scientific Research (NWO), GasDrive, is considering how to minimize emissions by combining LNG fueled prime movers. One part of the program being carried out by Delft University of Technology is focusing on the integration of a natural gas fueled solid-oxide fuel cell (SOFC) and internal combustion engine [ICEF2018-9615]. Anode-off gas from the SOFC would be supplied to the engine to enrich the natural gas with hydrogen. As a first step, the researchers examined the effect of hydrogen enrichment of natural gas on a marine natural gas engine. The hydrogen improved combustion stability and enabled better air/fuel ratio control and leaner mixtures to be used. An improvement in the BTE of the engine of 1.2% was noted while maintaining NOx at IMO Tier III values. The estimated efficiency improvement for the SOFC-ICE combination was 4%. They also found that hydrogen improved the load acceptance of the ICE and opened up the possibly of enabling a direct mechanical coupling of engine and propeller that provided additional efficiency gains through the avoidance of additional drive and electrical conversion inefficiencies.
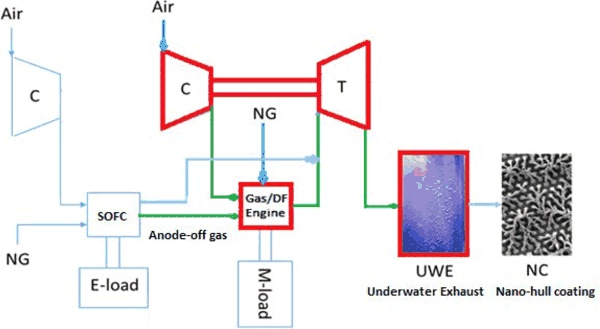
Japan’s Institute of Maritime, Port and Aviation Technology studied the fumigation of ammonia as a potential way to reduce CO2 emissions from marine engines [4672]. However, experimental work in a single cylinder engine revealed excessive emissions of NH3 and N2O, the latter gas being a potent climate warming agent. A diesel pilot injection used to assist with the low reactivity of ammonia fuel also showed some promise in reducing NH3 and N2O emissions.
Emission Control
The National Institute of Maritime, Port and Aviation Technology (Japan) investigated the effect of injection pressure on PM emissions and PM components—including elemental carbon (EC), organic carbon (OC), and sulfates—from a 6-cylinder, 750 kW at 1000 rpm, marine diesel engine [ICEF2018-9644]. Experiments were carried out using MDO fuel containing 0.048% sulfur. It was found that EC decreased with increasing injection pressure at both 25% and 50% load due to more complete combustion. On the other hand, OC increased with injection pressure at 25% load due to increased wall impingement. Hence, lowering injection pressure at lower loads would be beneficial for PM emissions.
Radio frequency (RF) sensors can be used to measure the soot load in diesel particulate filters, as well as other parameters in emission control catalysts, for instance the amount of ammonia stored in the SCR catalyst washcoat. CTS Corporation started the development of a simultaneous measurement of the PM load and NH3 inventory in an SCR-on-filter (SCRF) device using RF sensors [ICEF2018-9528]. Steady-state, constant temperature experiments were conducted in a bench reactor, using cordierite filter samples with a Cu-zeolite SCR catalyst coating. It was found that soot has a greater effect on the RF signal and can be measured in the presence of ammonia. However, the measurement of NH3 in the presence of soot is challenging. A neural network based method has been developed to statistically interpret data and calculate the NH3 load. For soot loads below 2 g/L, the sensor had an average ammonia measurement error of 0.011 g/L. Future work is planned to incorporate transient conditions and temperature compensation into the neural network training.
Researchers at Clemson University in South Carolina presented two papers on gasoline particulate filters (GPF). The first paper discussed an experimental investigation of the regeneration process in a catalyzed GPF [ICEF2018-9627]. Conducted experiments included loading of the GPF with soot, regeneration using a fuel cut-off strategy, and regeneration using secondary oxygen. The second presentation discussed the development of a thermal model for thermal protection control strategies for GPF health monitoring [ICEF2018-9687]. A ceria-washcoated GPF was used for the modeling work and for model validation.
* * *
Next year, the ASME Internal Combustion Engine Fall Technical Conference (ICEF 2019) will be held on October 20-23rd in Chicago, IL, United States.
Conference website: asme.org/events/icef